What are the water quality parameters being measured?
Salinity
Average ocean salinity is between 35 ppt (parts per thousand) and 36 ppt, (approximately 7 teaspoons in a liter of water). Oxygen availability depends on salinity: the higher the salinity level, the less dissolved oxygen (DO) is available. Salinity and temperature determine water density. Whereas the wind drives surface ocean currents, deep sea currents are carried by changes in water density. Since Earth’s climate is maintained by the ocean absorbing the excess heat from increasing global temperatures, climate change can alter water density and in turn, ocean circulation. To get an idea of what the very large and slow (1 000 years to complete a full circuit) thermohaline circulation looks like, here’s a video representing NASA‘s “Global Conveyor Belt”:
Measuring seawater salinity is necessary for calculating dissolved oxygen (DO) content, since the higher the salinity level, the less DO is available. The level of soil salinity also needs to be estimated for the restoration of banks and shores, because tolerance to salt differs among plant species. For instance, red mangroves are less salt tolerant than other mangrove species and thrive in 60-65 ppt soil salinity. Therefore, soil salinity can determine which restorative vegetation to plant.
Temperature
Like salinity, temperature affects density and DO. In fact, temperature affects most water quality parameters, including other dissolved gases, pH and conductivity. Temperature depends on seasonal factors: winter storms bring deeper uniform sea temperatures and the warmer months’ increased solar radiation heats surface waters that become less dense and float in a stable layer at the surface of the sea. Phytoplankton species proliferate and bloom in this sunny and warm surface seawater.
Organisms vary with temperature, and if this temperature changes, so can the diversity and number of organisms. Temperature equally influences the metabolism and biological activity of aquatic organisms (for example by inhibiting photosynthesis), and the toxicity of certain compounds, namely heavy metals and ammonia.

pH scale. Retrieved from http://www.ecofilms.com.au/aquaponics-health-understanding-ammonia-water-temperature-and-ph-balance/
pH
Low pH levels also favor the toxicity of heavy metals, and pH levels above 9 transform ammonium into dangerous, soluble ammonia. The pH scale ranges from 0 to 14. Below 7, pH is said to be acidic and pH greater than 7 is basic or alkaline. Pure water has a neutral pH of 7. To maintain fish communities, pH must range between 6.5 and 9, and most aquatic animals keep in the 6.5-8.0 pH range. Wastewater discharges can acidify seawater.
Dissolved oxygen
Dissolved oxygen (DO) is the oxygen available in natural waters for sustaining life. A water’s DO content indicates which aquatic organisms can survive in it, whether it is affected by waste oxidation and what capacities it has for self-purification. As mentioned above, DO depends on temperature and salinity, but also depth, clarity, sludge deposits, flow rate and time of day: in proximity of submerged aquatic plants, DO increases in the daytime as a result of photosynthesis, and decreases at night due to plant respiration.
DO enters the water either as an aquatic plant by-product or from the air, mixed in rapidly through aeration (waves, running water) or slowly diffused from the atmosphere. DO levels are 20% lower in seawater than in freshwater, and DO requirements for fish vary according to species. Swordfish alternate from shallow waters with DO concentrations of 7.7.mg/l to depths with DO concentrations of 2.5 mg/l. Around coral reefs, aeration and photosynthesis cause higher concentrations of DO, and many tropical fish thrive in such concentrations, usually ranging from 5 to 8 mg/l. On the other hand, oysters, mussels and clams need much lower 1 to 2 mg/l concentrations of DO, and crustaceans such as lobsters and crabs require DO levels between 1 and 4 mg/l.
Coastal fish will avoid seawater with less than a DO concentration of 3.5 mg/l, invertebrates move away from areas with DO concentrations lower than 2mg/l, and the survival rate of benthic organisms decreases when DO falls below 1 mg/l.
Turbidity
Turbidity is the measure (and the contrary) of water clarity. Water can become turbid for many reasons including erosion and debris, waste discharge, urban or agricultural run-off, algae and bottom feeders, to the point of affecting aquatic organisms. Less light penetration decreases photosynthesis and the production of DO. High turbidity increases the temperature of water (particles absorb heat) which also decreases the availability of DO.
When measured with a turbidity meter, turbidity is given in NTUs (Nephelometric Turbidity Unit). When using a Secchi disk, turbidity is reported according to how far light penetrates the water, in meters or JTUs (Jackson Turbidity Unit). However, water cloudiness also depends on flow and velocity. Therefore change in turbidity must be monitored over time from the same sampling point, to detect irregularities. To measure water clarity, scientists use a Secchi disk on a line with measurement markings.

IRetrieved from http://www.miseagrant.umich.edu/lessons/lessons/by-broad-concept/earth-science/water-quality/water-clarity/aragraph. Click here to add your own text and edit me. It's easy.
Nitrates and phosphates
Excessive amounts of nitrates and phosphates decrease DO, elevate temperature, and play on many other water quality indicators. These nutrients are essential for the survival of aquatic organisms, but in high quantities provoke eutrophication, which in turn alters plant and animal composition. Nitrate concentrations of 10 mg/l or higher are potentially toxic to warm-blooded animals, however for aquatic life, nitrates are the less toxic form of nitrogen. Occasionally, low nitrate levels will hide more dangerous concentrations of nitrites and ammonia that when suspected, should also be verified. Surface water contains less than 1mg/l of ammonia or nitrates, and waste water effluents reach levels of 30mg/l), thus nitrates efficiently indicate when water is soiled by sewage discharge or agricultural run-off.
Phosphates and nitrates share land-based sources. Natural sources, such as soil and rocks, are outnumbered by human sources, including sewage treatment plants and inadequate septic systems, agricultural and urban runoff, land development and drained wetlands.
Dissolved phosphorus passes from inorganic to organic, first absorbed by plants, then part of their tissues, then passed on through the aquatic food web. Bacteria convert the organic phosphorus deposited in the soil by plant and animal waste back into inorganic phosphorus, and the cycle starts over again. Phosphorus bind to soil particles and eventually settle, mostly downstream, as phosphate ions. Water quality tests investigate the presence of orthophosphates, one of the forms of inorganic phosphates. Even phosphate concentrations lower than 0.01 mg/l can have a serious impact on water quality.
Surfantants and fecal coliforms
Phosphates belong to the anionic class of surfactants (surface active agents), along with sulfates, sulfonates and carboxylates. These organic chemicals are used in a myriad of products and processes from soaps, lubricants and pesticides to wastewater treatment and petroleum recovery, despite their toxicity for marine life and their bioaccumulation. Surfactants in natural waters reveal land-based pollution, typically from inadequate sewage systems or coastal commercial activities.
Another water quality test that is undertaken to determine the source of nutrient overloads is a fecal coliform count. Usually not harmful themselves, coliform bacteria warn that other microorganisms living in animal and human digestive tracks that do carry diseases might be present. Sources of fecal contamination are faulty septic systems, animal feces, storm run-off and waste water treatment plants. Microbial source tracking can identify the origins of fecal contamination, PCR (polymerase chain reaction) genetically pointing to the fecal coliforms’ host.
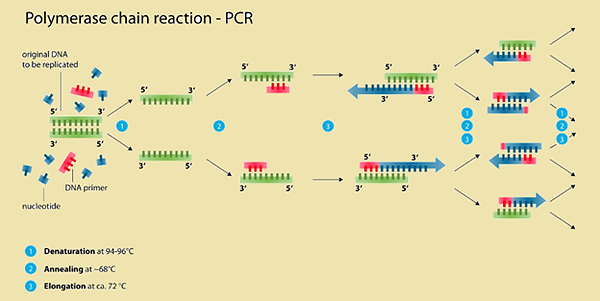
Retrieved from http://en.wikipedia.org/wiki/Polymerase_chain_reaction